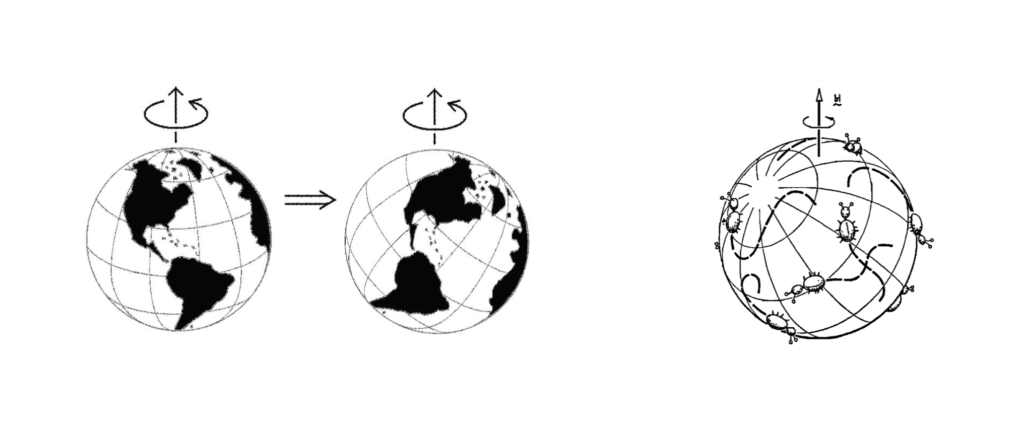
Not all [hotspots] who wander are lost (Exp391 Science Objectives, Part 2)
This post was written by Maya and edited by JR scientist Kevin Gaastra. Kevin is a fifth year PhD student studying tectonics and paleomagnetism at Rice University. When the landscape provides, he can be found snow skiing down some mountain in Tahoe.
When Professor Will Sager of the University of Houston announced that he would be going to Walvis Ridge to investigate true polar wander, a topic of of special significance, there was much talk and excitement within the scientific community.
The concept of true polar wander is scientifically rich, and somewhat peculiar, and has been a wonder of paleolatitude investigations for many years. But before we learn more about this fascinating concept, and see how it connects to the Walvis Ridge, we must begin again by visiting the Hawaiian islands and Emperor seamount chain.
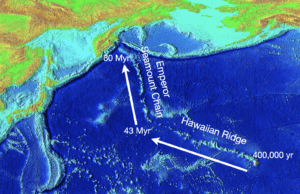
Due to the stark differences in their orientation, we refer to the geographic features as two separate groups: the Hawaiian islands and ridge, and the Emperor seamount chain. However, these chains of islands and underwater mountains are linked by more than proximity: the age progression that starts with the currently active volcanic island of Hawaii continues not just along the Hawaiian ridge, but also through each of the seamounts of the Emperor chain. This indicates that the same mantle plume formed both mountain chains, and therefore the same hotspot has been active in the Pacific since the formation of the first Emperor island 80 million years ago.
In the previous post, we talked about the general mechanism for the formation of hotspot tracks: a solid but fluid plume of hot, buoyant mantle material rises towards the crust where it melts the overlying rock to form an active volcano. The reason we do not see just one ever-growing volcano is that Earth’s surface is composed of several rigid sections, called tectonic plates, that move slowly. As a tectonic plate moves over the hotspot, the original volcano is carried away and a new one forms in its place.
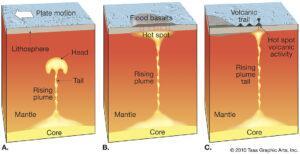
In theory, this is a very straightforward process, and one that can be a hoard of information about the movement of Earth’s tectonic plates and the convective flow of Earth’s mantle. The problem is that this hoard, like all hoards, comes with a dragon that makes things a bit complicated.
In this case, our dragon is the bend in the chain. What could have caused the volcanic islands to suddenly be carried off in a different direction? Perhaps the most straightforward explanation is that the Pacific Plate changed its direction of movement starting ~45-50 million years ago. It had originally been moving towards the north, but something caused it to change direction and head towards the west.
Though this explanation is simple, unfortunately not all the data collected from these rocks support this hypothesis. We must now delve deeper into the science, to understand a concept called paleolatitude. From Greek “palaios” meaning old, the term paleolatitude refers to the fact that, through the movement of Earth’s tectonic plates, many of the rocks on Earth’s surface are in a location that is different from where they formed. When scientists use analyses to determine where on Earth the rocks formed, they are determining paleolatitude.
There are several ways that scientists can determine the paleolatitude of geological formations. In the case of the Hawaiian-Emperor seamounts, scientists were able to study the signature of Earth’s magnetic field in the rocks to match them, much like one matches a puzzle piece, to the global map of field lines.
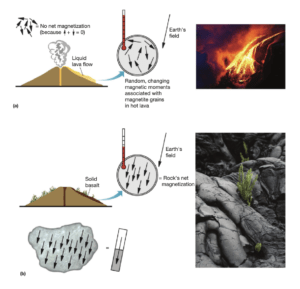
Here’s how it works: Many volcanic rocks have a small concentration of magnetic minerals inside them. Before the rocks solidify, when the lava is still liquid, the magnetic minerals are able to move around to align themselves with Earth’s magnetic field. This is similar to what it looks like when the needle of a compass orients itself. As the lava cools and hardens, the minerals become trapped in that orientation, preserving an image of what Earth’s magnetic field looks like in the area they formed. As long as the rock remains unaltered by remagnetizing processes (ex: melting, lightning strike), the magnetic minerals are preserved in the same orientation in which they formed, no matter how much that rock is transported by erosion or tectonic activity. When scientists perform complex analyses, they are able to interpret the magnetization of the rock and determine the latitude where it formed.
When paleolatitude measurements are used to reconstruct the tectonic motion of rocks from the Hawaiian-Emperor chain and surrounding tectonic plates, the paleolatitudes indicate that Hawaii must have moved south, despite the plate moving north.
Thus, a second hypothesis was proposed. Historically, geologists had hypothesized that mantle plumes were fixed in place under a moving plate, creating a stationary hotspot. What if instead the location of the hotspot changed over time? This concept became known as mantle plume migration.
Could a wandering hotspot be responsible for the unusual geography of the Hawaiian-Emperor chain? To answer this question, scientists developed detailed computer models to simulate all possible combinations of plate motion and hotspot migration that could have caused the bend in the chain. Though many different scientists modeled several different combinations of plate motion and plume migration, they struggled to find a solution that accurately mapped the movement in a way that agreed with the measured ages and paleolatitudes of the seamounts.
One more possibility can account for the morphology and paleolatitude measurements of these surprising seamounts: a phenomenon known as true polar wander. This is an idea that can be very hard to wrap your head around, so we’ll go slow. We’ll start with something basic: Earth is spinning. This is what gives us night and day and why we need time zones. Every spinning object has an axis, which is an invisible line that represents the center that the object is spinning around. Earth’s axis of rotation runs between the North Pole and the South Pole.
When an object is homogenous and rigid, there is nothing to affect the spin. However, if an object is NOT homogeneous, irregularities in its form can cause the spinning object to reorient around a new axis. The video below, created by NASA scientists, demonstrates the changing spin axis of a non-homogeneous rigid object.
Earth is also irregular. The surface of our planet is irregular, with mountains in some places and oceans in others. Middle Earth (in this case, the mantle) is also irregular: it is hot and buoyant in some places, and cooler and more dense in others. If an object is fluid, the internal motions of the fluid can change the object’s spin. Though the Earth’s mantle is solid, it flows like a liquid over millions of years and can alter Earth’s rotation.
This video, also created by NASA, first demonstrates the stable and consistent spin of rigid, more-or-less homogeneous objects. Beginning at 0:36, it is shown that the spin of fluid objects reorients over time.
Scientists have hypothesized that the physical irregularities moving in our planet have caused the entire surface to shift relative to its axis of spin. This would cause the paleolatitude of EVERYTHING to shift. Data indicate that the Hawaii-Emperor hotspot shifted ~12º southward. If true polar wander really did occur, we should expect to see a corresponding shift in other landmasses.
Conveniently, the Walvis Ridge is located just about on the opposite side of the world from the Hawaii-Emperor chain. If polar wander is truly the explanation for the confusing paleolatitude measurements of the Hawaiian and Emperor seamounts, then we can expect to see similar data in the Walvis Ridge: as the Hawaiian-Emperor chain shifted southward, the Walvis Ridge migrated toward the north.
This is what the scientists of IODP Expedition 391 are here to investigate. By collecting core samples from several locations along the Walvis Ridge, we can determine if crustal material on both sides of the Earth shifted comparably. If we see a corresponding paleolatitude shift to the north in rocks from the Walvis Ridge, and connect it to the shift to the south of the Hawaiian-Emperor chain, we can infer that there was a shift of all crustal material, which would indicate that true polar wander did occur.
And so, loading our drill bits and core barrels, we set off, seeking a path that would bring us to the underwater hills of Walvis Ridge, down into the land of hotspots.
Featured photo: Victor C. Tsai (left); Goldreich and Toomre, 1969 (right).